Premium Only Content
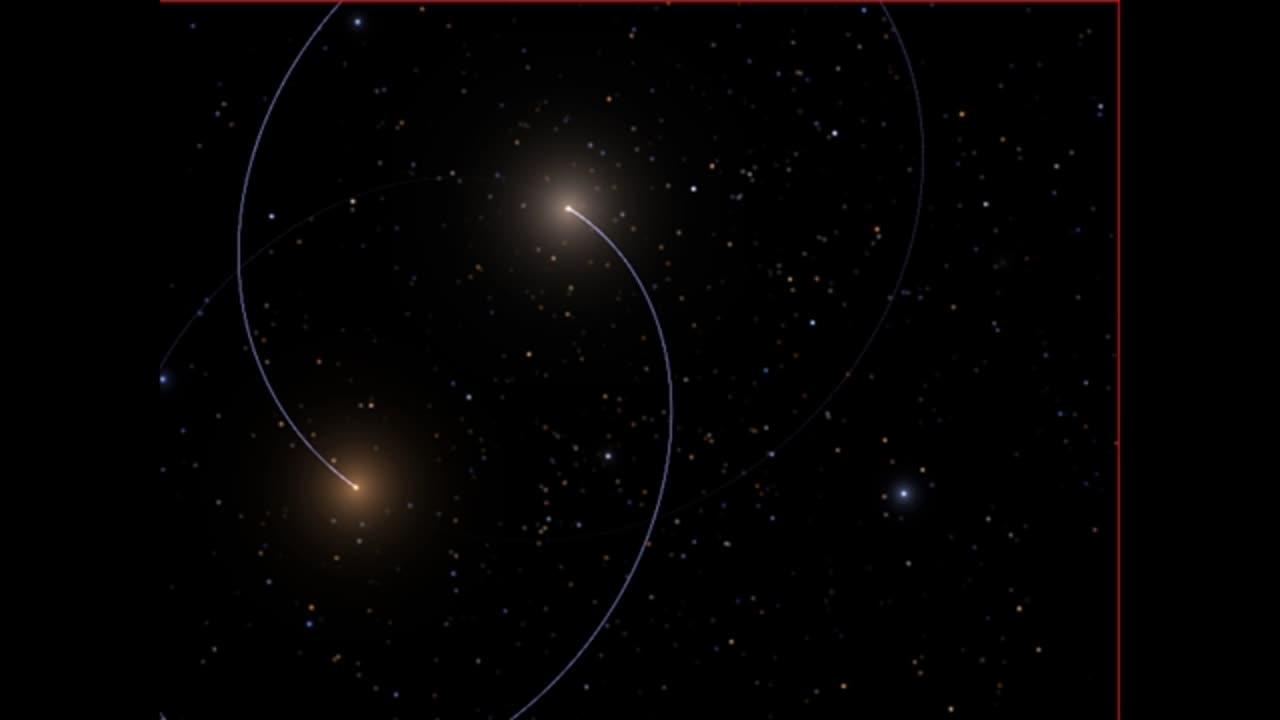
The ten Nearest G Stars. A Puke(TM) Audiobook
Index of Other Science Articles:
https://rumble.com/v3t4yzj-index-of-science.-music-by-dan-vasc.html
References
Stellar distances Parallaxes:
Gaia Data release 3, 2023
https://www.aanda.org/articles/aa/full_html/2023/06/aa43940-22/aa43940-22.html
Vallenari, and others.
Asterio seismology
Calculating asteroseismic diagrams for solar-like oscillations, 2011.
Timothy R. White
https://iopscience.iop.org/article/10.1088/0004-637X/743/2/161
Asteroseismology of Cool Stars, 2014.
http://www2.lowell.edu/workshops/coolstars18/articles/038-Huber_CS18.pdf
Daniel Huber
S Index:
The Mount Wilson observatory s-index of the sun, 2016
Luca Bertello and others
arXiv:1611.04540v2
Five Decades of Chromospheric Activity in 59 Sun-like Stars and New Maunder Minimum Candidate HD 166620, 2022.
Anna Baum, Howard Isaacson and others.
https://doi.org/10.3847/1538-3881/ac5683
S p h:
Statistics of BY Draconis Chromospheric Variable Stars.
Deepak Chalal, 2022
https://arxiv.org/abs/2206.05505v1
Photometric magnetic-activity metrics tested with the Sun: application to Kepler M dwarfs, 2014
Savita Mathur, J . Space Weather Space Clim. Volume 4, 2014.
https://www.swsc-journal.org/articles/swsc/full_html/2014/01/swsc130039/swsc130039.html
Rossby Number:
Helioseismic measurements of the Rossby number in the sun’s near-surface shear layer.
Greer 2016 and others, The Astrophysical Journal, 824, 2016 June 10
https://iopscience.iop.org/article/10.3847/0004-637X/824/1/4/pdf
The stellar activity-rotation relationship and the evolution of stellar dynamos.
Nicholas J. Wright, Gregory W. Henry and others, 2018
https://arxiv.org/abs/1109.4634v1
Hunting for anti-solar differentially rotating stars using the Rossby number, 2022
Q Noraz
https://arxiv.org/pdf/2208.12297
Stellar Coronal Mass Ejections
Martin Leitzinger and others, 2022
https://arxiv.org/abs/2212.09079
Implications of Recent Stellar Wind Measurements
Brian E. Wood, 2018
https://arxiv.org/abs/1809.01109v1
Debris Disks:
Maldonaldo 2012
Metallicity of solar-type stars with debris discs and planets
https://arxiv.org/pdf/1202.5884
Alpha Centauri
Coronal activity cycles in action X-rays from alpha Centauri A/B, 2016
https://arxiv.org/abs/1612.06570
A candidate short-period sub-Earth orbiting Proxima Centauri
J. P. Faria, 2022.
https://arxiv.org/abs/2202.05188
The Ups and Downs of Alpha Centauri, 2014
T. R. Ayres.
https://arxiv.org/abs/1401.0847
Coronal activity cycles in nearby G and K stars XMM-Newton monitoring of 61 Cygni and Alpha Centauri, 2021
F. Favata.
Observational Estimates for the Mass-Loss Rates of Alpha Centauri and Proxima Centauri Using HST Lyman-alpha Spectra, 2000
G.P. Zank and others.
https://arxiv.org/pdf/astro-ph/0011153
A new seismic analysis of Alpha Centauri, 2003
D. J. Montalban
https://arxiv.org/abs/astro-ph/0303467
Tau Ceti
Refining the Stellar Parameters of Tau Ceti, a Pole-on Solar Analog, 2023
Maria Korolik
https://arxiv.org/abs/2307.10394
Sigma Draconis
The Activity cycle of Sigma draconis, 1992
David Gray and Others.
https://www.researchgate.net/publication/234357409_The_activity_cycle_of_Sigma_Draconis
Asteroseismology of the Nearby K-Dwarf Sigma Draconis.
Marc Hon, and others 2024.
https://arxiv.org/abs/2407.21234
Eta Cassiopeiae
Stellar Diameters and Temperatures I. Main Sequence A, F, and G Stars
Boyajian and others, 2011
https://arxiv.org/abs/1112.3316v1
Fundamental stellar parameters for nearby visual binary stars. 1998
Fernandes and others.
https://www.researchgate.net/publication/234250185_Fundamental_stellar_parameters_for_nearby_visual_binary_stars_eta_Cas_XI_Boo_70_OPH_and_85_Peg_Helium_abundance_age_and_mixing_length_parameter_for_low_mass_stars
82 G. Eridani
Searching for the nature of stars with debris disks and planets, 2023
R de la Reza, and others.
https://arxiv.org/pdf/2302.01850
Delta Pavonis
Looking for Astrometric Signals below 20 meters per second: A Candidate Exo-Jupiter in delta Pavonis
Makarov, 2021
https://iopscience.iop.org/article/10.3847/2515-5172/abfec9
TARGET SELECTION FOR SETI
Turnbull 2003
https://iopscience.iop.org/article/10.1086/379320/pdf
Doppler Constraints on Planetary Companions to Nearby Sun-like Stars
Laliotis, and others 2023
https://iopscience.iop.org/article/10.3847/1538-3881/acc067/pdf
Xi Bootes
Fundamental stellar parameters of benchmark stars from CHARA interferometry
Karovicova, 2021
https://arxiv.org/abs/2109.06203
A SOPHIE RV search for giant planets around young nearby stars.
Grandjean 2021 and others.
https://arxiv.org/abs/2106.10754
Resolving the Xi Bootes Binary with Chandra,
Wood 2010
https://arxiv.org/abs/1005.3281
Morgenthaler 2010
Long-term magnetic field monitoring of the Sun-like star Xi Bootis A.
https://arxiv.org/pdf/1012.0198
Beta Hydri
Asteroseismic modelling of the solar-type subgiant star Beta Hydri
Brandao, 2018
https://arxiv.org/abs/1012.3872v1
Evolution of Solar-Type Activity: An Observational and Theoretical Perspective
Cuntz, 2023
https://arxiv.org/abs/2310.02798
Tess asteriosiesmology of Beta Hydri.
Metcalf 2024
https://arxiv.org/abs/2408.05482
Mu Cassiopeiae
Hubble Space Telescope Astrometry of the Metal-Poor Visual Binary Mu Cassiopeiae
https://arxiv.org/abs/2010.06609v1
Bond, and others 2020
Xi Urase Majoris
A T8.5 Brown Dwarf Member of the Xi Ursae Majoris System 2013
E.L. Wright and others
https://arxiv.org/abs/1203.5764v2
Beta Canum Venaticorum
Brandao 2018
One Light year equals 9.460730472580.8 Peta-Meters
HD128620, 128621 Alpha Centauri A, B
HD 10700, Tau Ceti.
HD 185144, Sigma Draconis,
HD 4614, Eta Cassiopeiae,
HD 20794, 82 Eridani,
HD 190248, Delta Pavonis
HD 131156, Xi Bootes
HD 2151, Beta Hydri
HD 6582, Mu Cassiopeiae
HD 98231, 98320, Xi Ursae Majoris
HD 109358, Beta Canum Venaticorum,
HD 1581, Zeta Tucanae
HD 115617, 61 Virginis
There are many stars within twenty light years of Sol, or equivalently, around Two hundred Peta-meters.
Stars can be classified using the Morgan-Keenan, or MK system, which is based upon the effective temperature, which is to say the equivalent temperature of a perfect black body radiating the same amount of electromagnetic energy. A G type star has an effective temperature of 5,300 to 6,000 degrees Kelvin. A cooler K star has an effective temperature in the range of 3,900 to 5,300 degrees, and the dimmer M star has an effective temperature from 2,300 to 3,900 K.
Most of the nearby stars are dim, convective and overly active M stars. Stars with the MK designation of G are relatively stable. Here we examine the nearest ten G dwarf stars, and their nature. Some of the stars in the list are considered as anchor stars, meaning stars of significant stability that they are examples for the Morgan-Keenan, MK classification system.
Typical G dwarf stars have masses of 80 to 104 percent of the sun, which is around two times ten to the thirty Kilograms. One Ronna ton is ten to the twenty seven tonnes, so the mass of the sun is around 2 Ronna tonnes.
The radii of most G stars is around 80 to 115 percent of the sun, or around zero point seven Giga-meters. Some of the stellar data can be precisely measured, such as orbital periods, and luminosities. Other data must be inferred, such as masses and radii, and there is agreement within experimental error of different observers.
The effective temperature is a factor of the bolometric luminosity and the stellar radius, and therefore the spectral type, “G” or “F” or “K” is not as an exact measurement as the color indices, and the differences in them, such as B minus V, or V minus R. The spectrum of a G zero dwarf star is shown, along with the Planck distribution for a blackbody of 5850 Kelvin. The real stellar spectrum, in red, is affected by many parameters, such as metallicity, gravity, rotation and internal turbulence. The Planck distribution is a function of only one free parameter, the temperature.
Many Astronomical values have significant uncertainties in them, and quite capable astronomers can disagree on exact values. As an example, consider the mass, age and logarithm of the surface gravity of Arcturus. These values are typical of early twenty-first century astronomy for nearby stars. Typical signal measurements in astronomy have high noise and thus the precision is far lower than most laboratory values.
As a further example, consider the radial velocity variations used to detect nearby planets. As the planet and the much more massive star rotate around a common barycenter, there is a variation in the motion of the star, which imparts a small Doppler shift on its spectral lines. Although the effect of the planet is systematic and continuous, with a well defined frequency, the actual contribution is very small.
In the figure, the radial velocity for Proxima Centauri D is shown. The effective radial velocity variation is 39 plus or minus 7 centimeters per second in the orbital velocity of the star, which is roughly the amplitude of periodic seismic vibrations in stars.
Sol.
The luminosity of Sol is relatively constant, and its effective temperature is 5,772 Kelvin.
The bolometric luminosity, L bol, is the total photon luminosity or energy radiated by the star. Typical stellar luminosities are in the range of ten to the 22 to ten to the 32 joules per second. For Sol, the luminosity is 3 point 8 times ten to the 26 watts. The logarithm to base ten of bolometric luminosity over four pi is 25.5. For example, at one terra-meter, ten to the twelve meters, log ten r squared is 24.
Therefore the flux per square meter is 25.5 minus 24, which equals one point five, or about 32 watts.
Sol has a mass of 1.9885 times ten to the 30 Kilograms, or 1.9885 Ronna tons. The log to base ten of the Cavendish gravitational constant G times Mass for the sun is 20.12. The quantity GM is often referred to using the Greek letter mu. At a distance of one Terra meter, log ten r squared is 24, and the effect of Sol’s gravitational acceleration is 20.12 minus 24 equals minus 3.88, or about 0.13 millimeters per second per second. If we rounded the Log of G M to 20, the result would be 0.1 millimeters per second per second, which is, by Astronomical accuracy, absolutely perfect.
The equatorial radius of Sol is 0.6957 Giga-meters, and its equatorial rotation period is 25.05 days.
Because the mass of the solar planets is non-negligible compared to the mass of the sun, the sun and the planets orbit around a common center of mass, or Barycenter. Relative to the center of the sun this is a measureable amount of deviation, which varies due to the different rotational periods of the planets.
There are various ways the stability of a star can be expressed.
A measure of the activity of the variability of the star is denoted as S P H, or the photospheric activity. This is the standard deviation of the luminosity curve over the average luminosity, averaged over five rotation periods. The sun rotates approximately once every 25 days, so multiple values of S P H for Sol are available. For Sol, the photospheric variability, S P H, values are in a range of around 67 to 315 parts per million. Taking an average over the years 1996 to 2008, S P H for Sol is related as 189.9 parts per million.
The solar cycle is considered to be caused by the oscillation of the magnetic fields of the sun, from a poloidal, or pole shaped, to a toroidal, or torus shaped mode.
Smaller, cooler stars of spectral type M or K exhibit a coupling between their rotating magnetic field and their chromosphere which result in quasiperiodic variations in intensity. This type of variable is named after the star B Y Draconis. The bolometric luminosity fluctuations for B Y Draconis stars are around 50 percent or about one half a magnitude.
For a “B-Y” Draconis type variable, the S P H value may range from one or five thousand parts per million, with two thousand parts per million, zero point two percent, being a typical value.
By comparison to the nearby stars, the Sun is quite stable, as shown in the figure, which compares stars of the suns temperature and hotter in terms of the S P H number.
Another parameter for describing the variability of a star is the Mount Wilson variability index, from O C Wilson, 1978. The Mount Wilson index is calculated using the emission of the Calcium two H and K lines, divided by the intensity of two nearby continuum bands, so that the resulting parameter is easily calculated, and insensitive to the shape of the continuum. The Mount Wilson index is a proxy indicator of stellar magnetic activity, and allows categorization of a star without the significant amount of observation time that the determination of the photosphere activity, which is the average over five rotational cycles, would require.
The Calcium two H band is located at 396.9 nanometers, and the K band is located at 393.4 nanometers.
The S M W index is also relatively indifferent to the use of raw or processed intensity values.
S M W is K plus H over the sum of the violet plus red pass bands. The H and K pass bands are 0.109 nanometer triangular bands, and the Red and Violet are 2 nanometer nearby continuum bands.
For Sol, the Mount Wilson Index has a mean over twenty three sun cycles of 0.1621.
Many Sol like stars have been examined over considerable periods of time. In 2022 Baum reported five decades of chromo-spheric activity for 59 Sun-like stars. It can be seen that “Sol” like stars have similar small variations in activity.
A closely related activity index, R H K is the relative intensity of the H and K calcium two lines, over the total flux, or R H K equals Flux K plus Flux H over sigma T effective to the fourth, where Sigma is the Stefan Boltzmann constant. As stated, R H K includes chromospheric and photospheric contributions. A derived quantity, R H K prime, has the photosphere contribution subtracted, however for active stars, the photosphere contribution is quite small. R H K is often quoted as a logarithm to base ten, often in units of ergs per square centimeter per second, equivalent to units of milli-Joules per square meter.
X ray luminosity is the energy of the star radiated in the x ray spectrum, which for sol is around 3 times ten to the nineteen Joules per second. A typical value of x ray luminosity is defined as the luminosity of x-rays in the region of 0.3 to 3.5 kilo electron volts.
The logarithm of the flare luminosity over the bolometric luminosity, Log L x over L bol for the sun is minus 6.24. The quantity L x over L bol is also called the X ray luminosity, and is often reported as the R x ratio. The ratio of Bolometric luminosity to flare luminosity is over one million, and by this definition we can classify the sun as relatively stable. For Sol, the range of X ray Luminosity varies from around 0.5 to 6 times ten to the 20 Watts, which is substantially more than the bolometric luminosity variations, which are around a few hundred parts per million.
The Rossby number, R zero, is a measure of the solar dynamo effect, and it is the ratio of the rotation period of the star to the time for convective mass turnover of a star. For example, M stars have a large amount of convective turn over, and an M star is usually fully convective so the Rossby number is small.
If the rotation period of the sun is taken as 25 days at the equator, and its Rossby number is around 1, then the time for the sun to convectively turnover is around 25 days. Other authors have suggested other values for the Rossby number of the sun, With Noraz in 2022 suggesting the accepted values for the Solar Rossby number as between 0.6 and 0.9. Greer in 2016 argued that the definition of the Rossby number, Flow velocity U over Length L and angular velocity Omega means that the Rossby number will necessarily be a variable dependent upon the scale length L. In that reference it was argued that the Rossby number is large at around five in the photosphere, and decreases to around 0.2 deep in the core.
Generally the Rossby number increases for small mass stars and it is an effective predictive parameter of the stellar dynamo effect, and hence solar flare activity.
The Rossby number for Proxima Centauri is 0.65, and the Rossby number of the highly convective Wolf 359 is 0.02.
Generally as the Rossby number R zero approaches 0.1, the X ray luminosity over bolometric luminosity approaches a saturated plateau value of ten to the minus three, or log minus three. Generally then, the stars with Rossby numbers of zero point one or less have a relative X-ray to bolometric luminosity a thousand times greater than Sol.
In his 2018 article, Nicholas Wright used 824 stars to fit a power law of R x equals L x over L bol is proportional to the Rossby number to the power of beta, where beta is around minus 2.70
Asteroseismology is the study of the oscillations of the stars. Since the star is a spherical mass of plasma and gas, it can pulsate and vibrate in various oscillatory modes, and can be modelled using spherical harmonics, Y l, n (Of theta and Phi). For an oscillating ball, a parameter delta nu is usually quoted, which is the difference in frequency of consecutive radial n modes, which have low angular l mode oscillation. As stars evolve, the convective envelope expands and the acoustic oscillation modes, p modes, or pressure modes, decrease in frequency. The other modes are known as gravity modes, or g modes.
Pressure modes are acoustic waves propagating through the compression and decompression of gas, and the pressure gradient acts as the restoring force. Gravity modes are pulsations due to the interplay of buoyancy and gravity, and buoyancy acts as the restoring force. Gravity modes are damped where convection is unstable, and are therefore usually confined to the deep interior for cool stars. Pressure modes propagate in radiative zones, and hence are more easily excited to observational amplitudes on the surface.
As a rule of thumb, delta nu is proportional to the cube root of the stars mean density.
For example a stellar density is more or less the square of the ratio of delta nu over delta nu of the sun, times the density of Sol. The figure from White 2011 shows an example of the accuracy of the density mode scaling law.
The deviation of the scaling law is generally a function of the stars effective temperature, as another figure from White, 2011 shows a relatively compact locus of points relating the effective temperature, density and mode frequency ratios.
Delta nu, the mode separation for Sol is 135 microseconds, or a period of 7,400 seconds. Its maximum pulsation frequency is at 3,100 microseconds, or a period of 322 seconds.
Another quantity indicative of the flare activity of a star is the rotational velocity of the surface of the star, v sine i. Because a star is generally inclined at some inclination angle, denoted as I, the sine of I is also included. For Sol, this quantity is 2 kilometers per second. For example, late type stars follow an approximation of x-ray luminosity L x is approximately proportional to the square of V sine i. Naturally, a star can have an orientation such that the inclination angle is very small, such as Tau Ceti, which has an inclination angle of seven plus or minus seven degrees.
B-Y Draconis variable stars will typically have rotational velocities around ten times higher than the sun, around 20 kilometers per second. The V sine I quantity, like the rotational period if measureable, is an characterization of the energy available to wind up the stellar magnetic dynamo, and produce sunspots, flares, and Coronal mass ejections.
Coronal mass ejections, CME’s, are an expulsion of partly ionized magnetized plasma, consisting of mainly hydrogen atoms, protons and electrons, into the solar heliosphere, or equivalently the stellar astrosphere.
CME’s on the Sun are known to be correlated to flares, and the correlation approaches 100 percent for energetic flares.
For Sol, the mass of material ejected from a CME is around ten to the twelve to ten to the fourteen Kilograms. Average velocities of solar wind are around 400 Kilometers per second. For Coronal mass ejections, cases of up to 3,000 kilometers per second recorded, for example in the years 1972 and 1989 for Sol. The mean kinetic energy of the flare particles are around ten to the 23 Joules, which is up to one percent of the solar luminosity.
Sol orbits around the Galactic Core, and the eccentricity of the orbit of Sol is 0.06 about the center of the Galaxy. The Local Standard of Rest, or LSR, is a circular orbit of the Sol galactic orbit radius, rotating about the galactic center. Sol is in motion relative to this LSR, since it has non-zero eccentricity. The velocity vector of Sol, U, V, W is 11.1, 12.2, 7.3 kilometers per second. The Co-ordinate system is chosen such that x is directed at the Galactic center, y in the direction of motion of the LSR, and z towards the Galactic north pole. Velocities of stars can also specified relative to the Sun, using the same U, V, W co-ordinate system. Using the Runge-Lenz vector, the velocities of the star, known as their proper motion, and its distance can be used to calculate the eccentricity of the orbit. The Runge Lenz vector allows the determination of the eccentricity and orbital parameters without the need to follow the star over a considerable amount of its orbit.
Stars with a large eccentricity relative to the Local Standard of Rest will travel through the spiral arms of the galaxy an experience a greater degree of collision with extra solar matter. Sol has a galactic year, or time of rotation around the galaxy of about 225 million years. Since Sol has an age of around four point six Giga years, Sol has rotated around the galaxy about 20 times.
There is considerable difference in the earlier values of the proper motion of stars, and hence the Space velocities calculated using the work of Holmberg 2007, and later is preferred.
The average galactic orbital eccentricities of the ten thousand stars of Holmberg in 2007 is 0.12 plus or minus 0.097. This means Sol has a lower than average eccentricity, and most nearby G stars have a higher than average galactic eccentricity.
This means that although the near stars are relatively near, they will not be over a lifetime of a Galactic year or more. Also, the environment of close approaches and perturbations of their planets will differ.
The stellar wind of a star removes angular momentum from a star, and it is a mechanism for reducing the stars rotation rate. Torque is measured in Force multiplied by distance, or units of energy, often quoted in ergs. For Sol, the solar torque can be estimated using various models as between 0.3 and 0.6 times ten to the thirty ergs, or from 3 to 6 times ten to the 22 Joules.
The age of the sun is approximately 4.6 Giga-years. Since one Giga-year is approximately 31.56 Peta-seconds, the Suns age is 142 Peta seconds. Just as Peta-meters are convenient metric units for near interstellar distances, Peta-seconds are convenient for stellar lifetimes. And Giga-meters and Giga seconds are convenient solar system units.
The bio-sphere, or region between which water is neither too cold or too hot, will depend upon the bolometric luminosity of the star. For the sun the biosphere range is 142 to 209 Giga-meters. Since this distance is defined in terms of temperature, it is solely determined by the stars bolometric luminosity.
The elemental composition is often described by the metallicity in dex, which is the logarithm to base ten of the concentration of Iron to Hydrogen, compared to Sol. For example, the Solar dex is 0.0, and a star with a ratio of ten times the Iron to hydrogen would have a dex value of plus one. A star with ten times less Iron to hydrogen would have a dex of minus one. Stellar metallicity is of interest in relation to debris disks and planets, and its correlation with orbiting matter is an area of active research. Maldonaldo in 2012 provided a series of histograms showing the tendency towards higher metallicity for stars with debris disks and planets.
All of these factors together determine a stars long term stability, and the stability of the environment around it. G stars typically have less convection and lower flare activity than M stars. This determines their level of biological interest.
In addition, stars which do not cross the spiral arms of the galaxy as they rotate, equivalently they have low galactic eccentricity, means their interstellar environment will not be widely varying.
Alpha Centauri.
The Alpha Centauri system consists of Alpha Centauri “A” and “B” rotating about a common barycenter, and Proxima Centauri, which is both a B-Y Draconis and UV Ceti flare star, distantly orbiting both.
Proxima Centauri is a cool “M” star with an effective temperature of around 3,000 plus or minus 50 Kelvin, and its variable star designation is 645 Centarus.
In addition to usual “B-Y” Draconis Variability, Proxima has exhibited super-flare type activity, with increases in the normal flux of up to forty times normal.
Alpha Centauri “A” has the Henry Draper, HD, catalog number 12-86-20, with “B” given the number HD 12-86-21. Their X-ray Luminosities, L x, are 2.2 times ten to the twenty watts, less than two thirds of Sol.
Alpha Centauri is located at a distance of 41.6 Peta meters from Sol. Since a Peta-meter, ten to the power of fifteen meters, or a thousand Tera-meters, is slightly less than a tenth of a light year, this distance is around 4 light years.
Alpha “A” is a G class star with a temperature around 5,790 Kelvin, whereas “B” is a cooler K star, with a temperature of around 5,200 K.
Alpha Centauri “A” is 50 percent more luminous than the Sun, whereas Alpha Centauri “B” has only half the luminosity of Sol. Through a telescope they are relatively easily resolved, and their orbits around their common barycenter has been determined.
The orbital eccentricity of “A” and B about their barycenter is 0.519, and the period is 79.762 years
Alpha “A” has of Mass of 2.15 Ronna tons, a Radius of 0.85 Giga-meters, and a Temperature of 5790 Kelvin. Its Iron over Hydrogen Metallicity is 0.2, its Log Luminosity over 4 pi is 25.66, and its Log mu is 20.16. It is very similar to Sol.
The age of Alpha Centauri “A” is 5.17 Giga years. Alpha Centauri “A” is also known as Gleise 559 “A” and HIP 71-6-83, among other names.
Alpha “A” rotates with a period of 28.3 days, and has a Bolometric luminosity over flare activity of x-ray luminosity of around one million, making it relatively stable.
The Stellar cycle for Alpha Centauri “A” is around 8.2 years, compared with the Solar 11 year cycle. The amplitude of the Luminosity variation of Alpha “A” is about half that of Sol.
The S index for Alpha “A” is around 0.16, compared to around 0.17 to 0.18 for Sol.
Since the S index depends upon the underlying continuum of the star. Subtracting the Photospheric component, the Log to base ten of R H K for the sun is minus 4.94, and minus 5.00 for Alpha Centauri “A”.
The Coronal mass ejection and mass loss rates for Alpha Centauri “A” are about half, possibly Zero point four six, of that of Sol. Alpha Centauri “A” has a delta nu for stellar oscillations of 105.73 microseconds, or a frequency of around 9,400 seconds, 2.6 hours. Its maximum oscillation frequency, nu max is 2,400 microseconds, or a period of 417 seconds.
Although dimmer than the cutoff for a G star designation, Alpha Centauri “B” is still close to Sol like.
Alpha Centauri B, also known as Toliman, is technically a K one dwarf star. It is smaller, less massive and cooler than Sol, with a slower rotation rate and higher metallicity.
Alpha Centauri “A” and B have, as of 2024, no confirmed planets. Proxima Centauri has one candidate, Proxima Centauri D, one confirmed planet, B and one unclear planet, Proxima C. Proxima “B” orbits with a period of 11.186 days, within Proxima B’s habitable biosphere, and C would have an orbital period of 1,928 days. The disputed Proxima D would orbit once every 5.122 days.
Proxima Centauri rotates around the Alpha barycenter once every 547 thousand years, with an orbital eccentricity of 0.5.
The U, V, W velocities of the Alpha Centauri system are -32, 3 and 12 kilometers per second, with a galactic eccentricity of 0.07.
Tau Ceti is a G 8 dwarf star, also known as GJ 71, HD 10-70-0, and HIP 81-02.
The Gia collaboration parallax for Tau Ceti is 3.652 plus or minus 3 milli parsecs, corresponding to a distance of 112.69 Peta meters.
Maria Korolik in 2023 gave the values of Radius 0.793 Sun, or 0.552 Giga-meters, and a Mass of 0.69 of Sol, or 1.37 Ronna tons. Baum’s 2023 value for the mass of Tau Ceti is 0.99 of Sol, and an age of 12.4 giga-years.
The age of Tau Ceti has been reported from 4.5 to over twelve Giga-years, and Korolik’s 2023 value is 10 Giga years.
Here, we can relate its rotational period is 46 days, its age is around ten Giga years, and its metallicity is minus zero point five dex. The effective temperature of Tau Ceti from Bioyajian 2013 is 5370 Kelvin, within experimental accuracy of the 5320 Kelvin value reported by Korolik.
From 1967 to 1995 The Mount Wilson Observatory obtained 1784 S index measurements, which are measurements of the Calcium two H and K absorption lines of Tau Ceti. The work of Baum published in 2022 gives an S med value for Tau Ceti of 0.169.
Astero-seismic oscillations of Tau Ceti were reported in Teixeira’s 2009 paper with a maximum power frequency of 4100 micro Hertz, and a standard deviation of 169 micro Hertz. Corresponding to an oscillation period of around 244 seconds. Its delta nu mode separation frequency was 148.5 microseconds. The logarithm of the chromospheric flux in the Calcium two H and K lines, R H K is minus 4.91, and the x-ray luminosity is about a twentieth of Sol, at 0.2 times ten to the 20 watts.
The upper limit of mass loss from Tau Ceti is estimated to be less than one tenth of that of Sol, suggesting weak stellar winds.
The inclination of the rotational axis of Tau Ceti to Sol is close to zero, with the estimates being seven plus or minus seven degrees. In 2014 Lawler reported measurements from the Herschel telescope array indicating that the debris disk of Tau Ceti has an inclination of 35, plus or minus ten degrees. The dust disk extends from around 1.5 Terra meters to 7.5 Terra-meters and has a mass of around one point two earth masses.
The Extreme precision Spectrograph, EXPRES, has a radial velocity, RV, resolving power of around 30 centimeters per second, and over 200 recent spectra, from 2019 onwards of Tau Ceti have been measured. Tau Ceti has four confirmed planets, Tau Ceti e, f, g, and h, as reported by Feng in 2017.
Tau Ceti’s galactic U, V, W velocity relative to Sol is 18, 29, 13 kilometers per second, with an eccentricity of 0.22.
Sigma Draconis, also known as GJ 764, HD 18-51-44, HIP 96-100, is a star classed as a K 0 dwarf, and re-classified in 2003 as a G9 dwarf, and so it is a borderline candidate for this list. The Gaia parallax from 2023 for Sigma Draconis is 173.4939 Milli-Arc Seconds, equating to a distance of 178 Peta-meters, or 18.799 light years.
Sigma Draconis is still often referred to as a K zero Dwarf star, for example in the Simbad data base, and in Marc Hon’s 2024 paper.
Sigma Draconis is an anchor star for the MK system. The effective temperature is in some cases reported as 5290 Kelvin, with a metallicity of minus 0.20 dex, and a mount Wilson activity index S M W of 0.189. Baum 2022 reports an S med value of 0.214.
Since Sigma Draconis has a temperature of around 5,300 Kelvin, just on the borderline of the definition of a G star. It has less metal that Sol, with a dex of minus zero point 23, and an age of three to four point five Giga-years. Baum in their 2023 paper gives the mass of sigma Draconis as 81 percent of Sol, with an age of 8.8 Giga-years. Marc Hon uses an age of 4.5 Giga-years for their calculations. The rotation period is close to that of Sol, with rotation periods reported as 20 to 26 days. The projected rotation rate, V sine eye is around 1.5 kilometers per second.
Sigma Draconis has very low variability, with a five to seven year stellar cycle.
The Log R H K is minus 4.81. The x-ray luminosity of Sigma Draconis is about eight percent higher than Sol, at 4.1 times ten to the twenty Watts. No known planets in 2024 are reported for Sigma Draconis.
The asterio-seismology of Sigma Draconis suggests its most powerful pulsation mode has a frequency of around 3700 micro Hertz, or a period of 270 seconds.
The space velocity vector relative to Sol of U, V, W of 36, 40 minus 10 kilometers per second, and its orbital eccentricity about the galaxy is 0.30.
Eta Cassiopeiae, GJ 34, HD 46-14, HIP 38 21, is also known as Achird. Eta Cassiopeia is located 183.7 Peta meters from Sol.
Eta Cassiopeiae is a binary star system, comprising of a G zero dwarf and a K 7 dwarf. The orbital period is 480 years, with an eccentricity of 0.497, and the separation range is 5.36 Terra meters to 15.8 Terra Meters. The orbit can be resolved by large telescopes.
Eta Cassiopeiae “A” has an effective temperature of around 6,000 Kelvin, which is at the extreme hot end of the range for G stars.
The age of Eta Cassiopeiae “A” is around 5.4 Giga years, and it has a lower metallicity than Sol, with a dex of minus zero point 31. Radial velocity and infrared measurements have ruled out large Jupiter sized planets.
Eta Cassiopeiae “A” has a mass and radius within 5 percent of Sol.
The X-ray luminosity of Eta Cassiopeiae “A” is smaller than Sol, at 2.6 times ten to the twenty watts. Log R H K is minus 4.94.
No known planets are reported for 2024.
Relative to Sol, the U, V, W space velocities are minus 29, minus 11, minus 17 kilometers per second,
With a galactic eccentricity of 0.07.
Eta Cassiopeiae B is a star of about half the size of Sol with about a sixteenth of its luminosity.
Eta Cassiopeia B is a cool K7 dwarf.
82 Eridani, GJ 139, HIP 15-5-10 and HD 20-79-4. Not to be confused with Epsilon Eridani, HD 220 49.
82 Eridani has had its stellar parameters studied by several authors, and these were compiled by Basant in 2022. 82 Eridani is a G 6 to 8 dwarf, also reported as a G 5 dwarf, with a temperature of 5401 Kelvin and a metallicity of minus 0.40 dex.
It has a mass of 0.813 of Sol, 1.614 Ronna tons, and a radius of 0.92 of Sol, or zero point six four Giga-meters. Its distance is 6.0414 parsecs, or 186 point four Peta-meters. Its logarithmic Bolometric Irradiance over four pi is 25.301, and the logarithm of the gravitational parameter mu is 20.03.
The biosphere of 82 Eridani lies between 115 to 169 Giga-meters, and its age is estimated from 6 to 13.5 Giga years, and its rotation period is 33.19 days. The projected v sine I of 82 G eridani is around 2 kilometers per second, comparable with Sol.
The x-ray luminosity of 82 G Eridani is 0.5 times ten to the twenty watts, about one eighth of Sol. Log R H K is minus 4.93.
82 G Eridani also has a confirmed dust disk. For comparison, the Kuiper belt disc of Sol is around 1.6 to 4.8 times the mass of the moon, or around 1.2 to 3.5 times ten to the 23 Kilograms. The mass of the 82G eridani dust belt is estimated as almost a million times less, at 5.2 times ten to the 17 kilograms. This would be equivalent to a unit density rock of 10.4 kilometers in diameter.
Radial velocity measurements of 82 Eridani have shown as many as six planets, four of which, b, c, d and e, are considered confirmed and reliable, and reported in the reference Feng 2017. Other orbiting bodies of smaller mass are not yet confirmed.
The latus rectum of the planets orbiting 82 Eridani are 13.6, 17.6, 32.7, 51, 69.7 and 130.6 Giga-meters.
The orbital periods of 82 G Eridani B, C, D and E are 18.3, 40.1, 90.3 and 147 days. For Comparison, the latus rectum of Mercury is 46 Giga-meters and of Venus is 107.5 Giga-meters.
The planet 82 Eridani six, or 82 Eridani “G” would therefore orbit with in the stars biosphere. The possible plant 82 Eriandi G may have an orbital period of 331 days, and a mass of 10.26 of Earth.
The orbit of 82 Eridani about the galactic center has an eccentricity of 0.42, and its Sol relative space velocity is minus 79, minus 93, minus 29 kilometers per second.
Delta Pavonis is also known as GJ 780, HD 19-02-48, and HIP 99-2-40.
Delta Pavonis is a G5 subgiant star, with a metallicity of plus zero point 33 and an effective temperature of 5571 Kelvin. It has an age estimate of 6.6 to 9.3 Giga-years, and a rotational period of 21.4 days. It is a suspected variable.
Delta Pavonis as a mass of 2.09 Ronna tons, a radius of 0.833 Giga-meters, a log irradiance of 25.577, and a log ten mu of 20.14. Due to its higher Luminosity than Sol, its Biosphere ranges from 157 to 232 Giga-meters. No known exoplanets, as of 2024, are confirmed for Delta Pavonis, although there is evidence from Laloitas 2023 suggesting a large planet of mass at least seventy Jupiter masses with a period of at least 37 years.
The coronal mass loss from Delta Pavonis is about ten times that of Sol, and it has more metal than sol, with a logarithmic dex of zero point 38, or more than double Sol.
Its logarithmic R H K is minus 4.97, and its x-ray flux is Lx is 1.8 times ten to the 20 Joules per second, less than half of Sol. The Mount Wilson Variability S is around 0.14, and the magnetic cycle is suggestive of a 17 year period.
Because of its high metallicity and low variability, Delta Pavonis is considered a prime target for old cat lady astronomers who believe in fantasies like SETI and Aliens.
Relative to Sol, Delta Pavonis Space velocities are minus 38, minus 17, plus 3 kilometers per second, with a galactic eccentricity of 0.11.
Xi Bootes is a double star system consisting of Xi Bootes “A” and “B”, located 22.03 light years, or 208 Peta-meters from Sol.
Xi Bootes “A” is a G 8 or 7 dwarf, while “B” is a K4 dwarf.
The Hipparcos catalog number is 72-6-59, HD 13-11-56, and its velocity relative to Sol is quite small, U, V, W of plus 6, plus two and zero kilometers per second, with a galactic orbital eccentricity of 0.07.
The orbital eccentricity of this system of Xu Bootes “A” and “B” about its common barycenter is 0.5117, with a rotational period of 151.505 years
Xi Bootes “A” is considered as a benchmark star.
Xi Bootes ”A” is a “B-Y” Draconis variable, and its axial rotation period is 6.2 days. The Solar cycle of Xi Bootes “A” is around 7.5 years, and its Rossby number, the ratio of rotation period to convective turnover is reported as around 0.35.
Xi Bootes “A” is a young star of age 200 mega-years, or 25 times younger than Sol, in fact its age is of the order of one Galactic year. It has a temperature of around 5,500 Kelvin and a metallicity of minus 0.1 dex.
Xi Bootes “A” has a mass of 1.750 Ronna Tons, a radius of 0.568 Giga-meters, a logarithmic irradiance over four pi of 25.233, and a log ten mu of 20.07.
Its biosphere is between 106 and 156 Giga-meters.
The Mass loss rate of Xi Bootes “A” is about the same as alpha Centauri “A”, which is about half that of Sol. Xi Bootes “A” has an x-ray fluence of 87.3 times ten to the twenty watts, compared with the value for Sol of 3.8. The Logarithmic R H K value is minus 4.32. The Chromosphere S index is quite large, ranging from about 0.38 to 0.45.
Xi Bootes “B” has a temperature of 4,350 Kelvin, and rotates once every 11.5 days.
The B companion has a mass of 1.312 Ronna tons, a radius of 0.424 Giga-meters, a logarithmic irradiance of 24.269, and a log ten mu of 19.914.
Because of its relative dimness, the biosphere of Xi Bootes B is between 36 and 52 Giga-metres.
The Xi Bootes system looses much more mass every year than Sol, by a factor of about 4.5.
Both the Xi Bootes stars are much younger than Sol, and rotate more than twice as fast. As of 2024, no planets are known for the Xi Bootes system.
Beta Hydri is a G 2 to zero sub giant of effective temperature 5872 Kelvin, with an age of 6.4 Giga years, and is considered a candidate for the future evolution of Sol. The sub giant classification indicates that Beta Hydri has exhausted its supply of hydrogen in its core, and is currently consuming helium, making it a marginal case for inclusion in this list of dwarf stars.
Beta Hydri is not to be confused with Beta Hydrae, HD 10-31-92.
Beta Hydri is also known as GJ 198, HIP 20-21 HD 21-51.
Beta Hydri has a mass of 2.148 Rona tons, a radius of 1.259 Giga Meters, a logarithmic irradiance over four pi of 26.027 and a log ten mu of 20.16. Its metallicity is minus zero point one dex, and its effective temperature is 5872 Kelvin. Based on TESS Data, the rotation period as reported by Metcalf in 2024 for berta Hydri is 23 plus or minus 2.8 days.
Since it is older than Sol by two Giga-years, its mass is ten percent higher and its luminosity greater than Sol by a factor of about three and a half, and is perhaps an example of solar evolution. Solar type oscillations have been studied by various authors for Beta Hydri. Its Mount Wilson variability S is around 0.14. The inclination of Beta Hydri to Sol is 55 plus or minus 17 degrees, and various authors have given the v sine eye of 2.2 to 4.3 kilometers per second.
Metcalf in 2024 identified seven radial, nine dipole and seven quadrupole solar-type oscillation modes. The maximum frequency was 1038 micro Hertz, or a period of 963 seconds. The Log R H prime for Beta Hydri is around minus 4.996. The X ray luminosity of Beta Hydri is reported as around 6.4 times ten to the twenty Watts, or ten to twenty times that of Sol. The Rossby Number, or Convective to rotational turnover rate for Beta Hydri is estimated as 0.91. In the same reference, Metcalf reported a Solar Rossby number of 2.33.
The biosphere of Beta Hydri extends from 245 to 390 Giga-meters. As of 2024, no planets are known for the Beta Hydri system.
Beta Hydri’s Galactic motion relative to Sol is -61, -46, -31 and its galactic eccentricity is 0.22.
The Mu Cassiopeiae system consists of a G type dwarf star, with a much lighter M4 dwarf.
Other names for this star are: GJ 53, Hip 53-36, HD 65-82 and Marfak West.
Mu Cassiopeiae has a U, V, W relative to Sol of minus 1, minus 127, minus 39 kilometers per second, and a galactic eccentricity of 0.48. This galactic motion means it is a star from the Galactic Halo.
The orbit of Mu Cassiopeiae B around Mu Cassiopeiae “A” can be resolved well from Sol, and several Hubble space telescope images are available. The orbital parameters of B around “A” are semi major axis 150 Giga-meters, or latus rectum 741 Giga-meters, eccentricity 0.5885, and orbital period 21.568 years. The inclination of the orbital plane of Mu “A” and “B” is around 110.67 plus or minus 0.06 degrees. Around Mu Cassiopeiae “A”, the companion “B” would swing from 441 to 1800 Giga meters in distance, and a planet in the biosphere of “A” would experience an extra illumination of “B” from 300 Giga meters to 1900 Giga meters distance.
Modern spectroscopic analyses of Mu Cas give a photospheric metal abundance of about one sixth that of the Sun, or a dex of around minus 0.83. The v sine eye of Mu Cassiopeiae “A” is 2.4 kilometers per second. The effective temperature is 5,306 Kelvin, although values from 5,300 to 5,600 have been reported. The logarithm of the chromospheric flux in the Calcium two H and K lines, R H K is minus 4.92, in units of ergs per second per square centimeter, or equivalently milli-Joules per square meter. The Mount Wilson S value of Mu Cassiopeiae is 0.157.
Mu Cassiopeiae “A” is smaller, less massive and less luminous than Sol. It has a mass of 1.479 Ronna tons, and a radius of 0.549 Giga-meters, a logarithmic irradiance of 25.132, and a log ten mu of 19.99. Its Biosphere has an extent from 94 to 139 Giga-meters. The Age of Mu Cassiopeia “A” has been stated at around 12 to 13 Giga-years, which would make it one of the oldest possible stars in existence.
Mu Cassiopeiae “B” has a mass of 0.344 Ronna Tons, a radius of zero point 202 Giga-meters, a logarithmic irradiance of 23.276 and a log ten mu of 19.36.
This much smaller dwarf would have a biosphere extent of 11 to 16 Giga-meters.
No planets are reported for the Mu Cassiopeiae system, as of 2024.
Xi Ursae Majoris, also known as Alula Australius, consists of two double stars orbiting each other, along with an additional brown dwarf, Xi ursae Majoris B C, of spectral type T 8.5. The five star group is located at a distance of 274 Peta meters from Sol.
Other names for this group are GJ 423 and HD 98 2 31 (“A”) 98 3 20 (“B”)
The U, V, W Proper motion of the star group is -2.4, -28.5, -20.3 kilometers per second, and its galactic eccentricity is 0.07.
The main components are an F 8 dwarf and G 2 dwarf, bound as RS Canum venaticorum variables, each with a stellar companion. RS Canum venaticorum variable stars are close binary stars that have active chromospheres.
The two double stars of Xi Ursae Majoris “A” and “B” orbit around each other with a period of 59.878 years, with an eccentricity of 0.398, with a semi major axis of 3.37 Tera-meters, or equivalently, a latus rectum of 2.84 Tera-meters. The two double stars can be resolved by earth telescopes. Each of the double star groups are not resolvable with current telescopes, and are so referred two as spectroscopic binaries. A spectroscopic binary has two stars close together, with relatively high velocities and the binary nature is inferred from the variation of the spectra due to Doppler shifts.
The two “A” group stars rotate around each other in about 22 months, or 669 days and have an eccentric orbit around their barycenter with an eccentricity of 0.53. The two B group stars rotate around each other with a period of 3.98 days. Usually, for example in the Perkins catalogue, the Xi Ursae Majoris “A” group is referred two as a G2 dwarf and a F 8.5 Dwarf.
Xi Ursae Majoris “A” “a” has a mass of 1.929 Ronna tons, and a radius of 0.710 Giga-meters. It has an effective temperature of 6005 Kelvin, a logarithmic irradiance of 25.567, and a log ten mu of 20.11.
Its slightly higher irradiance than Sol, produces a biosphere extending from 156 to 230 Giga-meters. Its effective temperature makes it a G zero dwarf star.
The companion red dwarf, Xi Ursae Majoris “A” B has a temperature of 3700 K, a mass of 0.756 Ronna tons, a radius of 0.223 Giga-meters.
The second group of the stars in Xi Ursae Majoris, “B” “A” and B B, rotate about each other in a roughly circular orbit, and the period of the red dwarf is 3.98 days, with a semi major axis of around 32 Giga meters, closer than the perihelion of Mercury about Sol of 46 Giga-meters.
Xi Ursae Majoris “B” “A” has a mass of 1.710 Ronna tons, and a radius of 0.640 Giga-meters. It has an effective temperature of 5692 Kelvin, a logarithmic irradiance of 25.381, and a log ten mu of 20.06. it is a Spectral type G 5 dwarf. In the Perkins catalog of MK stars, the Xi Majoris B group is often referred to a s a G2 Dwarf. The dex metallicity of B a is minus 0.35, indicating a metallicity of less than a half of Sol.
The companion red dwarf star, Xi Ursae Majoris “B” B has not been detected visually, and so independent measurements of its temperature, radius and Luminosity have not been published. Assuming a polytropic relation known as Lane’s law, we can expect the effective temperature of Xi Ursae Majoris B B to be around 3,100 Kelvin, and a radius of 119 Mega meters. Xi Ursae Majoris “B” B has a mass of 0.278 Ronna tons.
In addition to the four conventional stars, there is a brown dwarf Xi Ursae Majoris B C, of effective temperature around 600 Kelvin, and is located at a barycenter distance of around 600 Tera meters (4,000 Astronomical Units). Its spectral type is T 8.5, with the Wise number Wise J one-eleven eight thirty-eight point seventy, plus thirty one, twenty five thirty seven point nine-two. These Wise numbers are the ICRS J 2000 epoch Right Ascension, and Declination, respectively. The sub-solar metallicity and low chromospheric activity of Xi Ursae Majoris “A” argue that the system has an age of at least 2 Giga-years. The infrared luminosity and color of the brown dwarf suggests the mass of this companion ranges between 14 and 38 Jupiter Masses, 0.0266 and 0.072 Ronna Tons, for system ages of 2 and 8 Giga-years respectively.
Beta Canum Venaticorum, also known as Chara, Asterion, beta Cvn. In the Henry Draper catalog it is HD, 10 93 58 and in the Hipparchus catalog it is HIP 61 3 17. Asterion is a G zero Dwarf with an effective temperature 6,043 Kelvin, with a distance of 261 Peta meters from Sol. Its high temperature puts it on the extreme limit of being a G star and not the hotter class of “F” star.
Beta Canum Venaticorum has a mass of 1.929 Ronna tons, a radius 0.781 Giga meters, Logarithmic irradiance over four pi of 25.545, and a log ten mu of 20.11. It is within three percent of the mass and radius of Sol, and has a luminosity of 25 percent more than Sol.
Beta Canum has an age between 3.4 and 7.2 Giga-years, with less metal than Sol, with a dex of around minus zero point 2, or 60 percent of the metallicity of Sol.
Its Biosphere ranges from 152 to 224 Giga meters.
Beta Canum Venaticorum stability is such that it is considered a MK anchor star, or a representative star for stellar classification. It has previously been reported as a spectroscopic binary, in that a closely bound unresolvable companion was orbiting it. No current evidence seems available, and direct imaging has not resolved any brown dwarf companions.
Currently there are no known planets, and radial velocity measurements rule out anything larger than a Saturn sized planet closer than 10 Astronomical units, or 1.5 Terra-meters.
The Log R H K is minus 4.85 ergs per second per square centimeter, equivalently units of milli-joules per meter squared, with an x-ray luminosity of 0.6 times ten to the 20 Joules per second. The Mount Wilson S value of Beta Canum is 0.193.
Beta Canum Venaticorum has a U, V, W space velocity of minus 32, minus 4, 1.3 kilometers per second, and an eccentricity of 0.08, similar to the eccentricity of Sol at 0.06.
Two other stars are included from de Mello, and Ferndandez.
Zeta Tucanae, with the additional names of HD 15 81, HIP 15 99, GJ17, is an F 9.5 type Five Star, located at 265 Peta meters, or 28.01 light years from earth.
With a mass one percent less, a radius eight percent more, and a luminosity 25 percent greater than Sol, Zeta Tucanae is very similar to Beta canum Veniticorum.
Zeta Tucane has an effective temperature of 5970 degrees. Its biosphere extends from 159 to 234 Giga-meters. Zeta Tucanae is believed to be around half the age of Sol.
Zeta tucane is 2 to 3 Giga years old and has a dex of minus 0.18. Its rotation period is 31.1 days.
The Log R H K value of Zeta Tucane is minus 4.83 ergs per second per square centimeter.
The Current U, V, Z Galactic Space co-ordinates relative to Sol are minus 73, minus 3 and minus 43 kilometers per second, with an eccentricity of 0.19. This means that Zeta tucanae is moving at around 85 kilometers per second relative to Sol.
Like Beta Canum, Zeta Tucanae has a dust disk, and no known planets.
61 Virginis HD 11 56 17, HIP 64 9 24, GJ 506. 61 Virginis is a G 7 dwarf, located at a distance of 27.84 Light years, or 263.4 Peta-meters from Sol.
At present, three planets and a debris disc are believed to be present in the 61 Virginis system.
The planets “B”, C, and D have respective masses of 6, 198 and 11 times that of earth, with orbital periods of 4.2, 38 and 123 days.
The semi major axes are 7.5, 32 and 69 Giga-meters, inside of the innermost limit of the biosphere habitability zone, which is 128 to 189 Giga-meters for 61 Virginis. Large planets of Saturn size orbiting at 1 Terra meter have been ruled out. For comparison, since 61 Virginis is close to the luminosity of Sol, mercury orbits with a semi major axis of 59 Giga meters, with a sidereal period of 88 days.
61 Virginis has a mass within 1 percent of the sun, and a radius seven percent smaller, and a bolometric luminosity eighty-two percent of Sol.
It is older than the sun, with an estimated age of 6.1 to 6.6 Giga-years
Its Metallicity is minus 0.02, and it has a rotation period of 29 days, slightly longer than the 25.05 day axial rotation period of Sol.
The Log R H K value of 61 Virginis is minus 4.96. S med is reported as 0.164 in Baum 2022.
The space velocity of 61 Virginis is U, V, W is -23, -47, -31 kilometers per second, and its galactic eccentricity is 0.17.
References
Stellar distances Parallaxes:
Gaia Data release 3, 2023
https://www.aanda.org/articles/aa/full_html/2023/06/aa43940-22/aa43940-22.html
Vallenari, and others.
Asterio seismology
Calculating asteroseismic diagrams for solar-like oscillations, 2011.
Timothy R. White
https://iopscience.iop.org/article/10.1088/0004-637X/743/2/161
Asteroseismology of Cool Stars, 2014.
http://www2.lowell.edu/workshops/coolstars18/articles/038-Huber_CS18.pdf
Daniel Huber
S Index:
The Mount Wilson observatory s-index of the sun, 2016
Luca Bertello and others
arXiv:1611.04540v2
Five Decades of Chromospheric Activity in 59 Sun-like Stars and New Maunder Minimum Candidate HD 166620, 2022.
Anna Baum, Howard Isaacson and others.
https://doi.org/10.3847/1538-3881/ac5683
S p h:
Statistics of BY Draconis Chromospheric Variable Stars.
Deepak Chalal, 2022
https://arxiv.org/abs/2206.05505v1
Photometric magnetic-activity metrics tested with the Sun: application to Kepler M dwarfs, 2014
Savita Mathur, J . Space Weather Space Clim. Volume 4, 2014.
https://www.swsc-journal.org/articles/swsc/full_html/2014/01/swsc130039/swsc130039.html
Rossby Number:
Helioseismic measurements of the Rossby number in the sun’s near-surface shear layer.
Greer 2016 and others, The Astrophysical Journal, 824, 2016 June 10
https://iopscience.iop.org/article/10.3847/0004-637X/824/1/4/pdf
The stellar activity-rotation relationship and the evolution of stellar dynamos.
Nicholas J. Wright, Gregory W. Henry and others, 2018
https://arxiv.org/abs/1109.4634v1
Hunting for anti-solar differentially rotating stars using the Rossby number, 2022
Q Noraz
https://arxiv.org/pdf/2208.12297
Stellar Coronal Mass Ejections
Martin Leitzinger and others, 2022
https://arxiv.org/abs/2212.09079
Implications of Recent Stellar Wind Measurements
Brian E. Wood, 2018
https://arxiv.org/abs/1809.01109v1
Debris Disks:
Maldonaldo 2012
Metallicity of solar-type stars with debris discs and planets
https://arxiv.org/pdf/1202.5884
Alpha Centauri
Coronal activity cycles in action X-rays from alpha Centauri A/B, 2016
https://arxiv.org/abs/1612.06570
A candidate short-period sub-Earth orbiting Proxima Centauri
J. P. Faria, 2022.
https://arxiv.org/abs/2202.05188
The Ups and Downs of Alpha Centauri, 2014
T. R. Ayres.
https://arxiv.org/abs/1401.0847
Coronal activity cycles in nearby G and K stars XMM-Newton monitoring of 61 Cygni and Alpha Centauri, 2021
F. Favata.
Observational Estimates for the Mass-Loss Rates of Alpha Centauri and Proxima Centauri Using HST Lyman-alpha Spectra, 2000
G.P. Zank and others.
https://arxiv.org/pdf/astro-ph/0011153
A new seismic analysis of Alpha Centauri, 2003
D. J. Montalban
https://arxiv.org/abs/astro-ph/0303467
Tau Ceti
Refining the Stellar Parameters of Tau Ceti, a Pole-on Solar Analog, 2023
Maria Korolik
https://arxiv.org/abs/2307.10394
Sigma Draconis
The Activity cycle of Sigma draconis, 1992
David Gray and Others.
https://www.researchgate.net/publication/234357409_The_activity_cycle_of_Sigma_Draconis
Asteroseismology of the Nearby K-Dwarf Sigma Draconis.
Marc Hon, and others 2024.
https://arxiv.org/abs/2407.21234
Eta Cassiopeiae
Stellar Diameters and Temperatures I. Main Sequence A, F, and G Stars
Boyajian and others, 2011
https://arxiv.org/abs/1112.3316v1
Fundamental stellar parameters for nearby visual binary stars. 1998
Fernandes and others.
https://www.researchgate.net/publication/234250185_Fundamental_stellar_parameters_for_nearby_visual_binary_stars_eta_Cas_XI_Boo_70_OPH_and_85_Peg_Helium_abundance_age_and_mixing_length_parameter_for_low_mass_stars
82 G. Eridani
Searching for the nature of stars with debris disks and planets, 2023
R de la Reza, and others.
https://arxiv.org/pdf/2302.01850
Delta Pavonis
Looking for Astrometric Signals below 20 meters per second: A Candidate Exo-Jupiter in delta Pavonis
Makarov, 2021
https://iopscience.iop.org/article/10.3847/2515-5172/abfec9
TARGET SELECTION FOR SETI
Turnbull 2003
https://iopscience.iop.org/article/10.1086/379320/pdf
Doppler Constraints on Planetary Companions to Nearby Sun-like Stars
Laliotis, and others 2023
https://iopscience.iop.org/article/10.3847/1538-3881/acc067/pdf
Xi Bootes
Fundamental stellar parameters of benchmark stars from CHARA interferometry
Karovicova, 2021
https://arxiv.org/abs/2109.06203
A SOPHIE RV search for giant planets around young nearby stars.
Grandjean 2021 and others.
https://arxiv.org/abs/2106.10754
Resolving the Xi Bootes Binary with Chandra,
Wood 2010
https://arxiv.org/abs/1005.3281
Morgenthaler 2010
Long-term magnetic field monitoring of the Sun-like star Xi Bootis A.
https://arxiv.org/pdf/1012.0198
Beta Hydri
Asteroseismic modelling of the solar-type subgiant star Beta Hydri
Brandao, 2018
https://arxiv.org/abs/1012.3872v1
Evolution of Solar-Type Activity: An Observational and Theoretical Perspective
Cuntz, 2023
https://arxiv.org/abs/2310.02798
Tess asteriosiesmology of Beta Hydri.
Metcalf 2024
https://arxiv.org/abs/2408.05482
Mu Cassiopeiae
Hubble Space Telescope Astrometry of the Metal-Poor Visual Binary Mu Cassiopeiae
https://arxiv.org/abs/2010.06609v1
Bond, and others 2020
Xi Urase Majoris
A T8.5 Brown Dwarf Member of the Xi Ursae Majoris System 2013
E.L. Wright and others
https://arxiv.org/abs/1203.5764v2
Beta Canum Venaticorum
Brandao 2018
One Light year equals 9.460730472580.8 Peta-Meters
HD128620, 128621 Alpha Centauri A, B
HD 10700, Tau Ceti.
HD 185144, Sigma Draconis,
HD 4614, Eta Cassiopeiae,
HD 20794, 82 Eridani,
HD 190248, Delta Pavonis
HD 131156, Xi Bootes
HD 2151, Beta Hydri
HD 6582, Mu Cassiopeiae
HD 98231, 98320, Xi Ursae Majoris
HD 109358, Beta Canum Venaticorum,
HD 1581, Zeta Tucanae
HD 115617, 61 Virginis
-
8:13
PukeOnABook
2 days agoRahan. Episode 130 By Roger Lecureux. The Star Stone. A Puke (TM) Comic.
191 -
2:27:04
AirCondaTv Gaming
6 hours ago $7.75 earnedWar Thunder - Tankering Around for That 10 Bomb
37.5K4 -
4:19:05
SpartakusLIVE
9 hours agoThe MACHINE locks in for 12-hour POWER stream
30.7K1 -
1:58:40
Robert Gouveia
8 hours agoJ6 Coverup: Prosecute LIZ CHENEY; NY Judge REJECTS Immunity; Trump Breaks Gag?
140K70 -
2:22:06
WeAreChange
7 hours agoPSYOP Spreads: Drones Shut Down Airport In New York!
80.2K47 -
1:31:18
Redacted News
9 hours agoEMERGENCY! NATO AND CIA ASSASSINATE TOP RUSSIAN GENERAL, PUTIN VOWS IMMEDIATE RETALIATION | Redacted
231K432 -
56:45
VSiNLive
8 hours ago $5.73 earnedFollow the Money with Mitch Moss & Pauly Howard | Hour 1
79.7K2 -
52:44
Candace Show Podcast
8 hours agoMy Conversation with Only Fans Model Lilly Phillips | Candace Ep 122
93.5K335 -
LIVE
tacetmort3m
9 hours ago🔴 LIVE - RELIC HUNTING CONTINUES - INDIANA JONES AND THE GREAT CIRCLE - PART 5
326 watching -
26:52
Silver Dragons
7 hours agoCoin Appraisal GONE WRONG - Can I Finally Fool the Coin Experts?
35.6K2